.
O P E N A C C E S S S O U R C E : Frontiers in Oncolology
Radiation ulcers are a prevalent toxic side effect in patients receiving radiation therapy. At present, there is still no effective treatment for the complication. Senescent cells accumulate after radiation exposure, which can induce cell and tissue dysfunction. Here we demonstrate increased expression of p16 (a senescence biomarker) in human radiation ulcers after radiotherapy and radiation-induced persistent cell senescence in animal ulcer models. Furthermore, senescent cells secreted the senescence-associated secretory phenotype (SASP) and induced cell senescence in adjacent cells, which was alleviated by JAK inhibition. In addition, the clearance of senescent cells following treatment with a senolytics cocktail, Dasatinib plus Quercetin (DQ), mitigated radiation ulcers. Finally, DQ induced tumor cell apoptosis and enhanced radiosensitivity in representative CAL-27 and MCF-7 cell lines. Our results demonstrate that cell senescence is involved in the development of radiation ulcers and that elimination of senescent cells might be a viable strategy for patients with this condition.
Introduction
Radiation therapy is a common and efficacious treatment for patients with solid cancers. About 50% of cancer patients receive radiation therapy, alone or in combination with other treatment methods such as surgery (1). Among them, radiotherapy is the main treatment method for patients with head and neck tumors and has varying success (2), but oral mucositis is a crucial dose-limiting toxic effect (3). Radiotherapy is an important adjuvant treatment after surgery for breast cancer and can reduce the metastasis and mortality rates (4), but high-dose radiation exposure to superficial tissue ultimately leads to intractable skin ulcers. Although advances in radiotherapy such as dynamic intensity-modulated radiotherapy achieve precise delivery of radiation to cancer cells, side effects to surrounding tissues are still inevitable and bring great pain and/or cost to patients (5).
Various precautionary methods and therapies such as anti-inflammatory agents, local anesthetics, and growth factors have been used to treat painful ulcerations, but the clinical effects are poor (2). Palifermin, a recombinant human form of keratinocyte growth factor (KGF), is the only U.S. Food & Drug Administration–approved agent that is used to prevent oral mucositis in patients with bone marrow transplantation, but fibroblast growth factor receptor 2b (FGFR2b) is often overexpressed in cancer cells and increases the risk of tumor growth (6). Although hyperbaric oxygen therapy has been reported to reduce skin ulcers after radiation (7), the treatment duration is long. Therefore, the development of potential agents that mitigate radiation ulcers without accelerating tumor growth is intensively needed for oncological supportive care.
Cell senescence can be triggered by radiation-induced DNA damage and leads to delayed repair and regeneration of irradiated tissue (8). Persistent damage activates the cyclin-dependent kinase inhibitor p16Ink4a and causes cell cycle arrest (9). Cellular senescence is not just a state of proliferation inhibition and genetic alteration (10); senescent cells can secrete cytokines, called the senescence-associated secretory phenotype (SASP) including inflammatory factors (11, 12), tissue-reconstituted proteases, and growth factors, which can induce persistent chronic inflammation in the tissue microenvironment (13, 14) and promote cancer relapse (15). It has been reported that cordycepin and mammalian target of rapamycin inhibition can protect from radiation ulcers by inhibiting cell senescence (16, 17). These observations led us to explore if it is possible to mitigate radiation ulcers by eliminating senescent cells.
In this study, we show that senescent cells persist in radiation ulcers (clinical radiation ulcer samples and animal ulcer models), and clearance of senescent cells by the senolytics drug cocktail, dasatinib plus quercetin (DQ), can effectively mitigate radiation ulcers. Moreover, DQ treatment can enhance cancer cell radiosensitivity. Our findings suggest that cell senescence is involved in radiation ulcer development, and clearance of senescent cells can be a potential therapeutic method to mitigate radiation ulcers.
Materials and Methods
Human Skin Samples
Skin tissues were obtained from healthy volunteers and patients with breast cancer receiving radiation therapy from 2016 to 2018 at Hunan Cancer Hospital (the Affiliated Hospital of Xiangya School of Medicine of Central South University). Skin ulcer samples were obtained from the chest wall at the time of surgery and were processed for further analysis. The studies involving human participants were approved by the ethics committee of Hunan Cancer Hospital; the patients/participants provided their written informed consent to participate in our study.
Cell Culture
Human oral keratinocytes (HOK ATCC, PCS-200-014) were cultured in an oral keratinocyte medium containing antibiotics at 37°C in 5% CO2. Human fibroblasts, CAL27 (CRL-2095), and MCF-7 cells (ATCC, HTB-22) were cultured in Dulbecco's minimum essential medium with high sugar (Invitrogen) supplemented with 10% fetal bovine serum (Gibco) and 1% streptomycin/penicillin. The isolation protocol for human fibroblasts was described previously (18).
Conditioned Medium (CM)
CM was made by exposing young cells to a fresh medium for 24 h. SASP-CM was made by exposing senescent cells (7 days after radiation) to a fresh medium for 24 h. To collect (SASP+JAKi)-CM, senescent cells were treated with JAK inhibitor 1 (JAKi) or dimethyl sulfoxide (DMSO) for 72 h and cultured with a fresh medium containing JAKi or DMSO for another 24 h.
Animal Models
Female C3H mice (6–8 weeks) and male Sprague–Dawley rats (6–8 weeks) were purchased from Laboratory Animal Center of Army Medical University. To evaluate the effect of senolytics on radiation ulcers, animals were divided into non-radiation, radiation, and D+Q treatment groups. For local fractionated radiation, the head and neck area was exposed to irradiation at a dose of 6 Gy/day (X-RAD 160-225 instrument Precision X-Ray, 1.9 Gy/min) and treated with senolytics dasatinib (5 mg/kg) plus quercetin (50 mg/kg) (D+Q) (19, 20) by oral gavage every day for 5 days. Mice were sacrificed at days 3, 6, 8, and 10. For skin ulcer modeling, rats' right posterior limbs were exposed to a single dose of 40 Gy (0.9 Gy/min) radiation and treated with dasatinib (5 mg/kg) plus quercetin (50 mg/kg) (D+Q) by intraperitoneal injection every day for 5 days after irradiation. Rats were sacrificed at days 5, 8, 11, and 15 after irradiation.
Immunoanalysis and Histopathology
Tissues were fixed, embedded in paraffin, cut into 3-μm sections, and stained with hematoxylin and eosin (H&E). For p16 immunohistochemistry, slides were boiled in a citrate buffer for antigen retrieval after dehydration. Slides were then soaked in 10% hydrogen peroxide for 10 min to remove endogenous peroxidase and were washed. Slides were blocked in goat serum and incubated in primary antibody against p16 (Abcam, 1:100) at 4°C overnight. Washed slides were then incubated with secondary antibody for 40 min (biotinylated goat anti-rabbit IgG, BA-1000, Vector Labs), washed, and incubated in 3'-diaminobenzidine solution. For γ-H2AX and Ki67 immunofluorescence, antigen retrieval and blocking was performed as above, and primary antibody (γ-H2AX, Cell Signaling, 1:200; Ki67, Cell Signaling, 1:200) was applied and incubated at 4°C overnight. Slides were washed with phosphate-buffered saline and incubated with secondary antibody for 40 min (biotinylated goat antirabbit IgG, 594 nm) before adding an antifluorescence buffer containing 4′,6-diamidino-2-phenylindole for imaging.
Real-Time qPCR
Total RNA from tissues or cells was extracted using TRIzol (Life Technologies) and reverse-transcribed to cDNA using the Maxima First Strand cDNA Synthesis Kit (Thermo Scientific, K1671). Real-time PCR was performed by applying the SYBR Green (Takara) qPCR master mix following the manufacturer's protocol. ΔCt values were calculated as the following formula: ΔCt = Ct target – Ct actin. Values of sample reference to control were calculated using the ΔΔCT method; the difference of gene expression was calculated using the 2−(ΔΔCt) formula. qRT-PCR primer sequences are shown in Supplementary Table 1. Actin was used as an internal control.
SA-β-Gal Activity
Cells were seeded into 6-well plates and then either received 8-Gy (0.9 Gy/min) radiation or not. Cells were passed and assessed 7 days after radiation. SA-β-gal staining was done using a SA-β-gal staining kit (Cell Signaling) according to the manufacturer's instructions. First, 1 ml 4% paraformaldehyde was added to every plate to fix cells. Then, cells were incubated at 37°C for 24 h in a SA-β-gal staining solution (pH = 6.0, Cell Signaling). Blue-stained cells were senescent cells.
Flow Cytometry
Cells were seeded into 6-well plates at a density of 2 × 105 cells/well. Cells either exposed to radiation (8 Gy) or not were treated with DMSO or DQ (1 mM D+20 mM Q) for 24 h, digested with trypsin, and collected. Cells were then resuspended in a 100-μl binding buffer with 1-μl fluorescein isothiocyanate Annexin-V and 1-μl propidium iodine (PI; BD Biosciences, 556547). Finally, samples were analyzed by flow cytometry (C6, BD Biosciences, San Jose, CA). For cell cycle analysis, cells were fixed with Fixation/Permeabilization Diluent/Concentrate (eBioscience) for 30 min. Subsequently, intracellular Ki-67 (eBioscience) and Hoechst33342 (Sigma) staining were performed using PermWash solution (eBioscience). Cells were washed once prior to flow cytometry analysis.
Western Blot
Cells were extracted in a cell lysis buffer (Cell Signaling) with protease inhibitors (Sigma). Proteins were loaded into each lane on a 5–12% gradient sodium dodecyl sulfate/polyacrylamide gel and transferred to immunoblot polyvinylidene fluoride membranes (Bio-Rad). Membranes were blocked with 5% skim milk and probed with primary antibodies at 4°C overnight. Horseradish peroxidase-conjugated secondary antibodies (Beyotime) were applied for 1 h at room temperature. The band intensities were visualized and quantified using an enhanced chemiluminescence detection system (Bio-Rad Laboratories). Primary antibodies used were as follows: poly ADP-ribose polymerase (PARP, 1:1,000, abcam), caspase 3 (1:1,000, abcam), cleaved caspase 3 (1:1,000, abcam), p-JAK1(1:1,000, abcam), p-JAK2 (1:1,000, abcam), and β-actin (1:1,000, Beyotime).
Enzyme-Linked Immunosorbent Assay (ELISA)
The concentrations of human inflammatory cytokines from HOK and fibroblasts cell supernatant were measured with ELISA kits. IL-1α (KE00123), IL-6 (KE00139), IL-1β (KE00021), IL-8 (KE00006), and tumor necrosis factor (TNF)-α (KE00154) ELISA kits from ProteinTech were used following the manufacturer's protocols. Generating a linear standard curve based on the OD value of the standard, the expression of protein was calculated using the formula generated above.
Statistical Analysis
Comparisons between two groups were analyzed using unpaired Student's t-tests, and values are presented as mean with SD. Statistical significance was set as *P < 0.05, **P < 0.01, and ***P < 0.001. SPSS 13.0 statistical software was used to perform all statistical analyses, and GraphPad Prism 7.0 was used to generate graphs.
Results
Senescence Biomarkers Accumulate in Human Radiation Ulcer After Radiotherapy
Senescence can be induced by multiple mechanisms such as DNA damage, reactive oxygen species (ROS) production, and oxidative stress (21), and DNA damage is a critical mediator of cellular alterations caused by radiation exposure (22). To explore the hypothesis that cell senescence and SASP are related to human radiation ulcers after radiotherapy, we first analyzed established senescence genes in the GSE103412 dataset (23) corresponding to mucositis in patients with tonsil squamous cell carcinoma (during and after radiation therapy) and control human cohorts (healthy mucosa and patients before radiotherapy). CDKN2A (p16) and TP53 were upregulated within oral mucosa samples of individuals with mucositis during and after radiation therapy (Figure 1A). In addition, HIST1H3B, HIST1H2BM, HIST1H3C, HIST1H3H, HIST1H1A, HIST1H4D, and HIST1H1B were downregulated (Figure 1A) in mucositis samples, especially at day 7 after radiation. This is notable since histone gene expression downregulation is a response to DNA damage (24). Ki67 (a marker of proliferation) was downregulated, indicating that radiation decreased the proliferative capacity of mucosa. Based on the hypothesis that senescent cells promote the development of radiation ulcers through the secretome, we analyzed the expression of SASP genes in human mucositis transcriptome datasets (GSE103412). Expression of pregnancy-associated plasma protein A (23), several matrix metalloproteinases (MMPs), and interleukin (IL) family members were also increased after radiation therapy (Figure 1A).
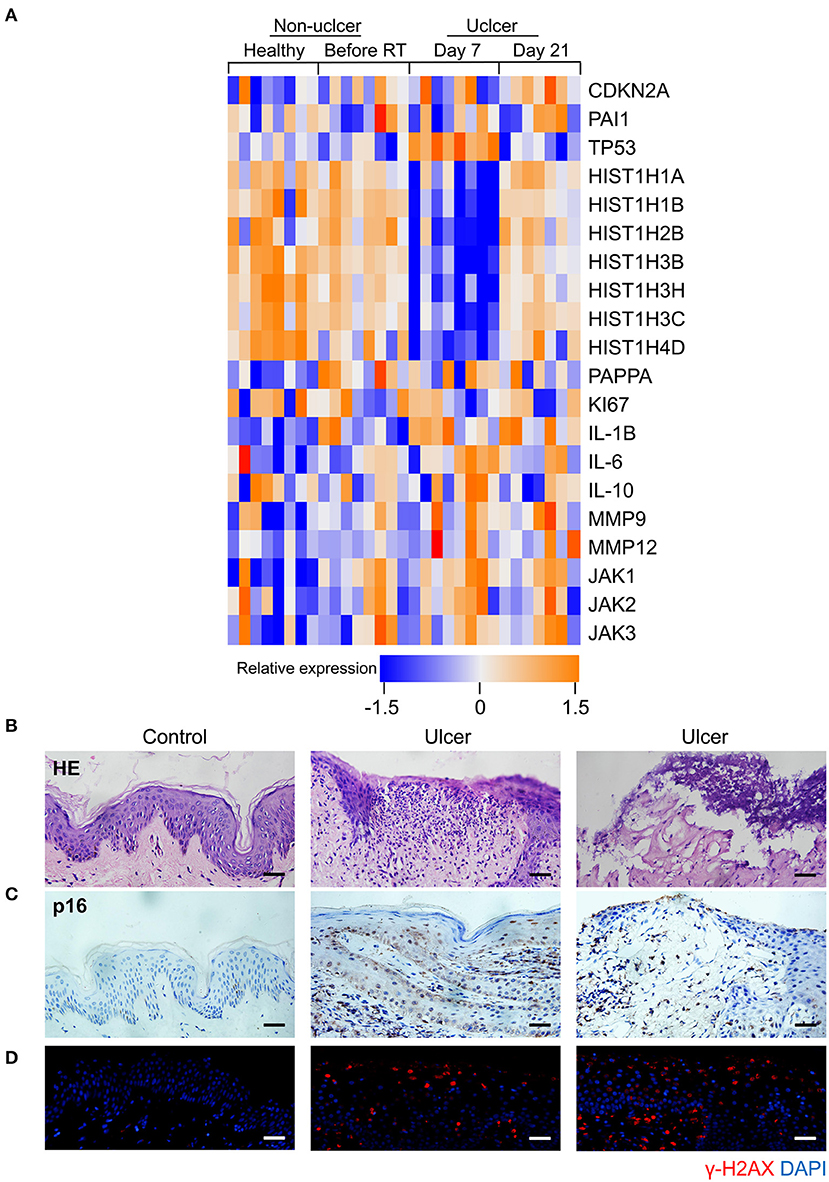
Figure 1. Senescence biomarkers accumulate in human radiation ulcer after radiotherapy. (A) Heat map showed the expression of senescence, DNA damage, and SASP genes in mucositis in patients with tonsil squamous cell carcinoma (during and after radiation therapy) and control (healthy mucosa and patient before radiotherapy) human cohorts (healthy n = 8, before radiation n = 8, day 7 n = 8, day 21 n = 7). (B) Histological analysis of skin tissues from healthy volunteers and radiotherapy patients. © Immunohistochemistry staining of p16 of skin tissues from healthy volunteer and radiotherapy patients. (D) Immunofluorescence staining of γ-H2AX of skin tissues from healthy volunteer and radiotherapy patients. (B–D) Healthy n = 1, radiotherapy patients n = 4, skin tissue from the chest wall; scale bar, 50 μm.
We also immunohistochemically detected p16 and γ-H2AX in skin tissue samples from healthy volunteers and patients with breast cancer receiving radiation therapy. As shown in Figure 1B, a lack of epithelium in the tissue was observed in ulcer tissue samples compared to normal skin. We also found a remarkable increase in the senescence marker p16 (Figure 1C) and the DNA damage marker γ-H2AX (Figure 1D). Collectively, our results indicate that senescence biomarkers accumulate in human radiation ulcers after radiotherapy, and senescence may play a critical role in promoting human radiation ulcers.
Radiation Induces Persistent Cell Senescence in Animal Ulcer Models
To further confirm the correlation between radiation ulcers and cell senescence, a mouse oral ulcer and rat skin ulcer model were established (Figure 2A). For radiation-induced oral ulcers, the head and neck of mice were treated with fractionated radiation of a 6-Gy dose/day for 5 days (other body parts were covered with a lead board). Mice were euthanized at days 3, 6, 8, and 10, and the tongues were removed and analyzed. For radiation-induced skin ulcer, each rat's right posterior limb was exposed to a single 40-Gy radiation under anesthesia (25). As shown in Figures 2B,C, the irradiated tongues and skin exhibited severe destruction of the epithelial layer compared to normal epithelial morphology. Furthermore, both models showed increased immunohistochemical staining for the senescence marker p16 at different time points (Figure 2D). qRT-PCR showed that senescence markers p16, p21, and plasminogen activator inhibitor-1 (PAI-1) were increased in irradiated mice/rats (Figures 2E,F). We found that the SASP factors (26) [IL-1β, IL-6, IL-1α, IL-8, IL-10, TNF-α, MMP3, MMP12, and monocyte chemoattractant protein-1 (MCP1)] were all significantly upregulated in irradiated tongue and skin tissues compared to non-irradiated controls (Figures 2E,F). These results indicate that senescent cells and the SASP persist in radiation ulcer. These results are consistent with previously reported data for senescence-associated beta-galactosidase (SA-β-gal), a known marker of senescent cells (16). Therefore, eliminating senescent cells might be a viable strategy to alleviate radiation ulcers.
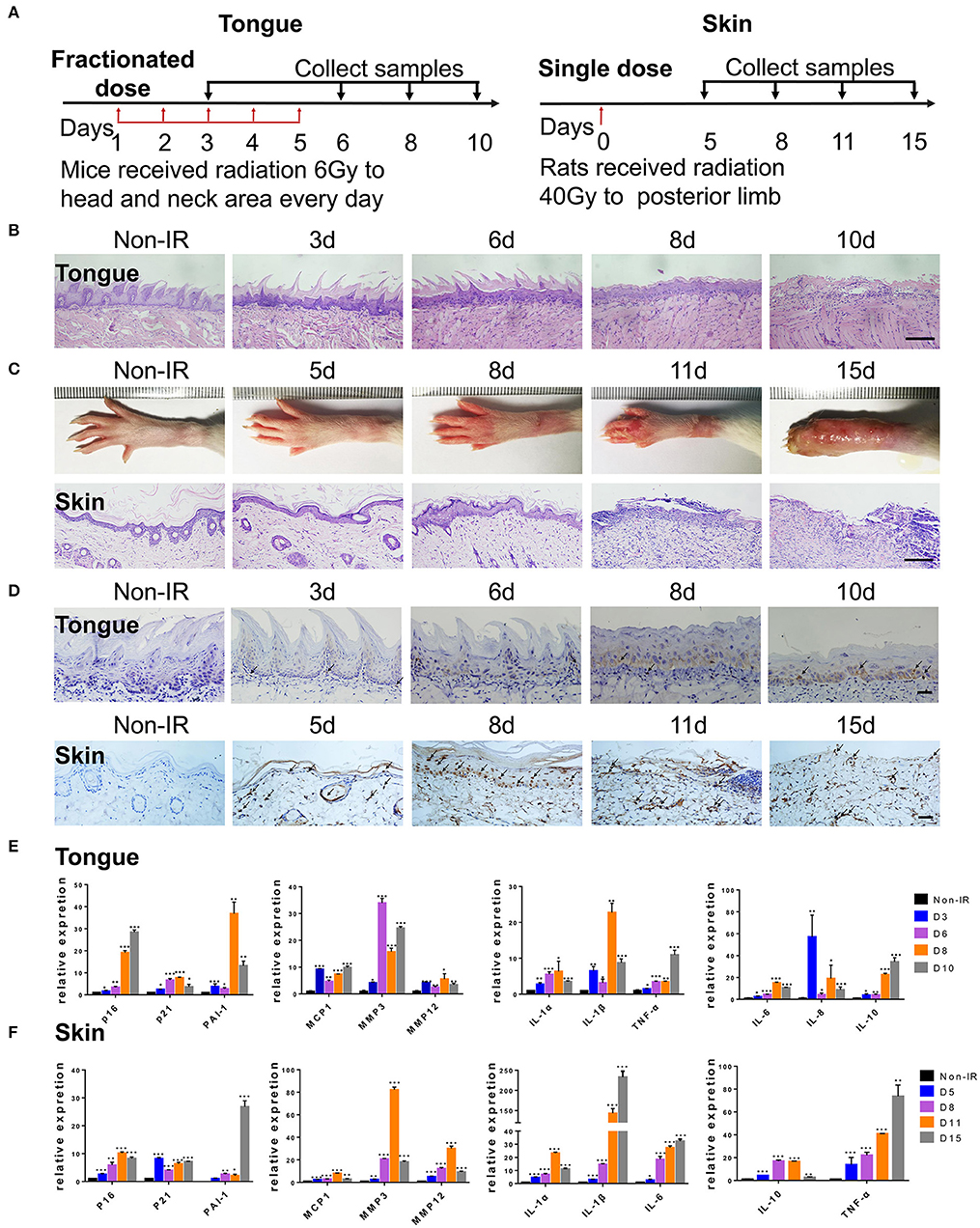
Figure 2. Radiation induces persistent cell senescence in animal ulcer models. (A) Radiation and drug treatment scheme for mice (left) and rats (right). (B) Histological analysis of mouse tongue tissues 0–10 days postradiation (n = 3). © Representative images of hind limb 0–15 days postradiation (top); histological analysis of rat skin tissues 0–15 days postradiation (bottom) (n = 3). (D) Immunohistochemistry of p16 in mouse tongue and rat skin tissues (n = 3). As indicated by the arrow, brown represents positive cells. (E,F) The expressions of p16, p21, PAI-1, and SASP genes (IL-1α, IL-10, IL-1β, TNF-α, IL-6, MMP3, IL-8, MMP12, and MCP1) in different time points were quantified by qRT-PCR (mean with SD; n = 3, *P < 0.05, **P < 0.01, ***P < 0.001; Student's t-test). (B,D) Scale bar, 100 μm; © scale bar, 50 μm.
.../...
.