.
Abstract
The aging process starts directly after birth and lasts for the entire lifespan; it manifests itself with a decline in an organism’s ability to adapt and is linked to the development of age-related diseases that eventually lead to premature death. This review aims to explore how microRNAs (miRNAs) are involved in skin functioning and aging. Recent evidence has suggested that miRNAs regulate all aspects of cutaneous biogenesis, functionality, and aging. It has been noted that some miRNAs were down-regulated in long-lived individuals, such as let-7, miR-17, and miR-34 (known as longevity-related miRNAs). They are conserved in humans and presumably promote lifespan prolongation; conversely, they are up-regulated in age-related diseases, like cancers. The analysis of the age-associated cutaneous miRNAs revealed the increased expression of miR-130, miR-138, and miR-181a/b in keratinocytes during replicative senescence. These miRNAs affected cell proliferation pathways via targeting the p63 and Sirtuin 1 mRNAs. Notably, miR-181a was also implicated in skin immunosenescence, represented by the Langerhans cells. Dermal fibroblasts also expressed increased the levels of the biomarkers of aging that affect telomere maintenance and all phases of the cellular life cycle, such as let-7, miR-23a-3p, 34a-5p, miR-125a, miR-181a-5p, and miR-221/222-3p. Among them, the miR-34 family, stimulated by ultraviolet B irradiation, deteriorates collagen in the extracellular matrix due to the activation of the matrix metalloproteinases and thereby potentiates wrinkle formation. In addition to the pro-aging effects of miRNAs, the plausible antiaging activity of miR-146a that antagonized the UVA-induced inhibition of proliferation and suppressed aging-related genes (e.g., p21WAF-1, p16, and p53) through targeting Smad4 has also been noticed. Nevertheless, the role of miRNAs in skin aging is still not fully elucidated and needs to be further discovered and explained.
1. Introduction
The aging process starts directly after birth and lasts through the entire lifespan. For the majority of the population, it becomes a pressing problem around age 40–60 when the middle-age period of life free of diseases is followed by the period associated with the beginning of age-related diseases (ARDs) that eventually leads to a shortening of life. There are numerous theories describing the causative effects that deteriorate health, and almost all of them are based on the mechanisms that affect homeostasis at the genetic, molecular, cellular, tissue, and organismal levels. Many theories suggest that the appearance of various aging phenotypes occurs because of the age-dependent accumulation of damages [1]. At the same time, some individuals demonstrate the delayed development of aging features or ARDs and have a significantly longer life span despite their socioeconomic status.
An increase in life expectancy around the world is positive, but it is a double-edged sword. On the one side, older people have more opportunities to live an active lifestyle, stay with their families, or continue to work and contribute to multiple areas of society. The extent of this possibility mainly depends on health. On the other side, an increased longevity is directly associated with a higher odds of developing ARDs. Therefore, this means that long-lived people will suffer from pain and disability for a much more extended period of time due to degenerative processes and ARDs (cardiovascular, diabetes, cancer, Parkinson’s, Alzheimer’s, and others). These morbidities drastically complicate a patient’s life and hasten mortality. It is worth noting that clinical manifestations of the abovementioned ARDs are preceded by long (10–20 years) asymptomatic periods of illness development [2]. If no changes are implemented, these trends will continue, and aging will become a significant social and economic burden [3]. The increased life spans have created a pressing need for a better understanding of the pathogenesis of the aging process and the identification of biomarkers and possible therapeutic targets for ARDs.
We know that human longevity is determined by multiple factors, including genetic and environmental influences. About a quarter of the changes in our genetic material over our life period are due to various mutations or epigenetic regulation [4]. The results of epidemiological studies show that centenarians usually demonstrate features of healthy aging, accompanied by the lack or the delayed onset of ARDs. The process of aging is associated with various physiological and pathological molecular mechanisms, including intricate patterns of changes in gene expression leading to changes in the performance of multiple cell types, tissues, and organs. The insulated genetic aberrations in aging-relevant pathways trigger segmental, tissue-selective aging phenotypes [5]. Nevertheless, for a majority of tissues, it remains unknown which age-related modifications play a prominent etiological role in the mechanism of aging, or which ones are just epiphenomena [6].
The most evident and visible symptoms of aging in humans are manifested by changing skin appearance due to continuous exposure to exogenous irritants. Skin is the first line of defense against abiotic and biotic environmental factors [7,8]. Despite an ongoing renewal process, the cutaneous regenerative capacity reduces with age. A decrease in regenerative potential is often linked to a decline in the elimination of senescent cells, and their gradual accumulation results in the physiological aging of the tissue itself [9]. Many questions remain as to how to achieve longevity and healthy aging [10]. Skin seems to be a perfect health sensor because an alteration in its appearance directly signals the ongoing pathological changes in the organism. Thus, research on dermal health and aging is essential for an overall understanding of the processes of aging.
Current the biology of aging includes studies that explore or combine the issues of genomic instability, telomere length, deterioration of mitochondrial function, cellular senescence, epigenetic modifications, proteostasis, exhaustion of stem cells, autophagy, altered intercellular communication, as well as the deteriorated sensing accuracy or trophic function [1,11,12].
A typical signature of aging is the down-regulation of the expression of proteins involved in the electron transport chain. It remains disputable whether this is a reason or an outcome of the aging process. The respiratory chain is controlled by the communication between mitochondria and the nucleus, and changes in the expression of mitochondrial genes depend on energetic demand and a reductive load [13]. A disruption or reductive overload of the respiratory chain leads to the overproduction and release of reactive oxygen species (ROS) or Ca2+ by mitochondria or elevated levels of AMP and ADP. Signals of the abovementioned mitochondrial dysfunction are sensed by the mammalian target of rapamycin (mTOR) or AMP-dependent protein kinase (AMPK) and calmodulin. Subsequently, these signals are transduced to PPARγ-coactivator 1α (PGC1A), which activates the switch in the nuclear mitochondrial biogenic program [14,15]. Dysfunction in these pathways has been described in association with age-related human pathologies and has been observed in aged tissues [13]. Nevertheless, AMPK/mTOR and PGC1A are not unique pathways involved in the regulation of mitochondrial and nuclear interactions in the functionality of the respiratory chain but also require the cellular redox state via Sirtuin 1 (SIRT1) and c-myc. It was found that the SIRT1 expression and nicotinamide adenine dinucleotide (NAD+) levels decrease throughout aging in murine skeletal muscle and lead to dysfunction in mitochondrial biogenesis activation via SIRT1 and c-myc in the AMPK/PGC1A-independent fashion [16].
The majority of genetics-based longevity studies are primarily focused on sirtuins SIRT1 and SIRT3 and calorie restriction. Sirtuins have been reported to contribute to the extension of lifespan due to the improvement of mitochondrial function during dietary restrictions [17,18]. Additionally, sirtuins SIRT1, SIRT6, and SIRT7 are involved in regulating the telomere length and integrity via deacetylase activity, which also facilitates chromatin condensation and histone modification [19,20]. The potential mechanism might include the TOR signaling pathway.
It is intriguing that within the last decade, it was discovered that microRNAs (miRNAs) were widely involved in the modulation of almost all processes in an organism, including aging. The potential role of miRNAs in aging biology is linked to the regulation of the expression of proteins maintaining the insulin-like growth factor (IGF)-1 and TOR signaling pathways [21]. Moreover, publications show that microRNAs play an essential role in regulating the balance between cellular proliferative capacity and replicative senescence [22]. The expression of various miRNAs was found to be directly associated with lifespan duration, ARDs, or their predisposition. Therefore, the expression of a subset of miRNAs also might be considered as one of the aging markers and as a predictor of chronological age, longevity and premature aging, the development of pathologies, or even a risk of mortality [23]. Unfortunately, there is a deficiency of robust information about miRNA functions in the processes of human aging and longevity, and many of these aspects still need to be discovered [24].
To date, there is a lack of information about the relation between miRNA involvement in the dermal and organismal aging process from the perspective of longevity and presumably skin disorders. Furthermore, there is currently a paucity of data that demonstrate possible links between miRNA expression and aging or age-related clinical outcomes [25]. In this review, we will focus on associations between miRNA expression and organismal and cutaneous aging.
2. Skin Structure and Biology
Skin is the outermost organ and the largest one in the human body. It occupies about 8% of the total body mass of an adult human, covering 1.8 m2 of surface area [26]. Apart from being a protective barrier, the skin is also responsible for maintaining homeostasis, including the prevention of the percutaneous loss of electrolytes, fluid, and proteins; it also controls immune activity and sensory perception as well as temperature regulation [27,28,29]. The skin consists of three separate but functionally interdependent layers: the epidermis, dermis, and hypodermis (Figure 1). Their cellular components maintain the mechanical defense, photoprotection, immunosurveillance, nutrient metabolism, repair, and rejuvenation [30,31].
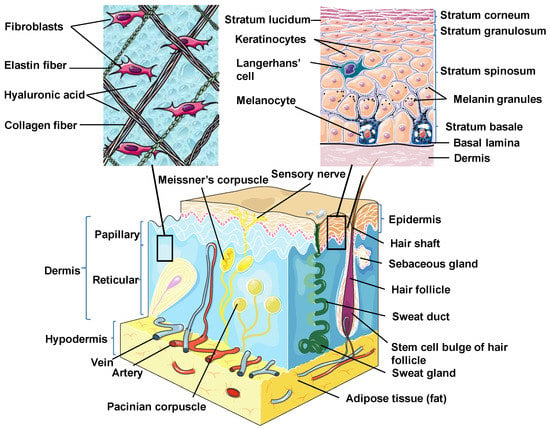
Figure 1. Skin structure. Schematic diagram showing the structural components of the normal human skin. This figure was created using images from Servier Medical Art Commons Attribution 3.0 Unported License (http://smart.servier.com). Servier Medical Art by Servier is licensed under a Creative Commons Attribution 3.0 Unported License.
2.1. Epidermis
The epidermis is the outermost layer of the skin derived from the ectoderm and is tightly interlocked with dermal ridges. It mainly serves as a protective bulwark between the outside world and the internal environment of the body. Other epidermal functions are related to immunoprotection, thermoregulation, ultraviolet protection, resistance to trauma, and the maintenance of energy metabolism. The epidermis consists of keratinocytes, Langerhans cells, melanocytes, neuroendocrine (Merkel) cells, and inflammatory cells (Figure 1) [32]. Keratinocytes comprise over 90% of the cellular population of the epidermis and are connected by desmosomes and tight junctions. They produce and store an intracellular fibrous protein—keratin—that provides hardness and water-resistant properties to the skin and its appendages (hair and nails) [33]. Langerhans cells resemble macrophages in their functions and perform phagocytic activity. Melanocytes produce the pigment melanin that gives hair and skin its color and is also involved in UVR damage protection. Merkel cells function as a touch-perception receptor [32].
Depending on the location in the body, the skin is classified into two types: thin skin and thick skin. They are respectively made of four or five layers of epithelial cells in the epidermis. Thin skin consists of the following layers or strata (from the innermost layer to the outermost one): the stratum basale (the basal layer or stratum germinativum) which contains stem cells that attach the epidermis to the basal lamina; stratum spinosum (the spinous or prickle cell layer); stratum granulosum (the granular cell layer); stratum corneum (the cornified cell layer) (Figure 1). The fifth layer, located between the stratum corneum and the stratum granulosum, is known as the stratum lucidum (the clear cell layer), found predominantly in thick skin which covers the palms of the hands and the soles of the feet [31,33].
2.2. Dermis
The dermal layer delivers nutrients and supports the circulation of the skin [34]. It is separated from the epidermis by a structurally and chemically complex basement membrane zone presented by intertwining collagen fibers. The dermal-epidermal interface is tightly interlocked and stabilized by hemidesmosomes [33]. Structurally, the dermis is a connective tissue layer composed of an interconnected net of collagenous and elastin fibers embedded within the ground substance produced by fibroblasts; it is abundant with lymph and haemocirculation, nerves, and additional structural components (hair follicles and sweat glands). The majority of dermis cells are presented by fibroblasts that possess multifunctional activity but that are predominantly involved in the production of extracellular matrix (ECM) components [31]. Apart from fibroblasts, the dermal layer is composed of a broad number of endothelial and neural cells, supporting elements as well as mast cells embedded within a matrix of collagen and glycosaminoglycans. In the dermis, there are also dendritic and nondendritic monocyte/macrophages, myofibroblasts, along with dermal dendrocytes expressing factor XIIIa that are implicated in the hemostatic and inflammatory processes [35,36].
The dermis consists of two layers. The first one is a papillary layer, composed of loose areolar connective tissue with fibroblasts, collagen bundles, and thin elastic fibers that form a loose mesh and provide a mechanical anchorage and nutrients to the overlying epidermis. Additionally, it contains phagocytes, lymphatic capillaries, nerve fibers, and touch receptors known as the Meissner corpuscles [33]. The second layer is a reticular layer, which is a thicker layer located between the papillary dermis and the subcutaneous adipose tissue. It is made up of irregular dense connective tissue with thick collagen bundles and coarse elastic fibers and is supported by wide vascularization and the sensory and sympathetic nerve supply. Elastin fibers in this layer render the dermal elasticity and movements. While collagen fibers maintain the structural and tensile robustness, they are also responsible for the support of skin hydration by binding water. They are widespread in the papillary compartment and hypodermal layer [31].
2.3. Hypodermis
The hypodermis (also called the subcutaneous layer of the skin or superficial fascia) is a layer directly below the dermis which transitions to the fascial layers. It consists of well-vascularized, loose, areolar connective tissue with a lesser collagen content and abundant adipose tissue that form a layer of variable thickness depending on its location in the body. The hypodermis facilitates cutaneous mobility. The adipose tissue serves as a storage of metabolic energy and provides thermal insulation, cushioning properties, and shock absorption for the integumentary system [31,33].
About 30,000 dermal cells die every minute. At the same time, cutaneous development and normal physiology is a highly adapted process that depends on the cooperation between genetic networks and various regulatory factors [37]. Despite daily exposure to environmental damage, the skin sustains continuous self-renewal to replace old or injured cells and repair the damaged tissue [38]. However, when pathogenic effects exceed the protective cutaneous capabilities or regenerative properties depleted with age, it potentiates cellular senescence and different dermatological diseases [39].
3. Molecular Aspects of Skin Development
Cutaneous formation begins within the first two weeks of development. The ectoderm eventually forms the epidermis and melanocytes as well as the nervous system. The latter one starts developing during the third week of fetal life, when the ectoderm creates the neural plate within it and initiates neural crest development. The mesoderm gives rise to blood vessels, muscles, bones, and fibroblasts, except for some subpopulations that are derived from the neural crest of ectoderm. Besides this, the endoderm is not involved in cutaneous formation [32]. It is believed that neural crest cells secrete the Wnt1 ligand, a signaling molecule that activates the transcription factor and cytoskeletal protein, β-catenin. The latter controls the epithelial differentiation and the functioning of stem cells and appendages. Fibroblast growth factors induce the neural fate, while bone morphogenetic proteins (BMPs) and WNT signaling regulate the epidermal fate [38,40].
3.1. The Development of Epidermis
The surface ectoderm stimulates the generation of a single layer of basal keratinocytes from the germinativum—a layer of cuboidal undifferentiated and mitotically active cells. Keratinocytes are famous for an abundant synthesis of intermediate filaments keratins. Germinativum expresses the gene p63, which is essential for epidermal differentiation. Experiments demonstrate mice lethality immediately following birth due to p63 deficiency [41]. In humans, TP63 mutation causes several autosomal dominant ectodermal dysplasias, which are characterized by different combinations of limb, ectodermal, and orofacial abnormalities as well as alopecia, suggesting the role of p63 in maintaining stem cell proliferation [42,43].
Numerous signaling pathways control the epidermis formation and stratification. For instance, the ligand Delta or Jagged binds the receptor Notch, which initiates transcription and epidermal differentiation. The inhibition of the Notch pathway results in the deficiency of the development of the cutaneous barrier [44]. The epidermis fails to stratify in cases with a low level of expression of the p63 transcription factor, which consequently deteriorates appendage formation [45]. The development of hair follicles and epidermal interfollicular lineages is regulated by the notch 1 and 2 signaling pathways [46]. The mitogen-activated protein kinases (MAPK) signal transduction pathway (also known as the Ras-Raf-MEK-ERK pathway) likewise regulates the epidermal proliferation and differentiation. The deletion of its key enzyme Mek1/2 (mitogen-activated protein dual kinases 1/2) results in cutaneous underdevelopment [47]. Conversely, the augmented activity of the epidermal growth factor (EGF) involved in the MAPK pathway potentiates proliferation and epidermal tumor growth [48].
Three distinct pools of stem cells are located in the interfollicular epidermis, the bulge, and the sebaceous gland supporting epidermal homeostasis. Skin stem cells undergo continuous self-renewal and differentiation into the required cell lineages to replenish cells such as keratinocytes, which die due to programmed termination or injury [28]. The renewal of the cutaneous barrier occurs via the spinous transition of basal cells in mature skin in parallel with changes in gene expression (e.g., the down-regulation of Keratin5/14 and up-regulation of Keratin1/10) controlled by p63 and the canonical Notch pathway [45].
3.2. The Development of Dermis
The dermis is formed from the mesodermal layer. The distinct boundary between the epidermis and the dermis appears at the eighth week of gestation. Fibroblasts represent the primary cell type that compose the dermis and demonstrate the ability to regulate the epithelial cell function. They also abundantly secrete collagens and other extracellular matrix molecules. In addition, fibroblasts show a high level of heterogeneity depending on the skin location. Thus, early embryonic dermal fibroblast progenitors can potentially differentiate into several cell types. For instance, the upper dermal fibroblast progenitor cells (PDGFRa, Blimp1, Dlk−, Irig1 markers) become the dermal papillae (a ball of fibroblasts that control hair keratinocytes) and the arrector pili muscle (the muscle attached to small hairs that causes goosebumps) [49,50]. In the upper dermis, there are papillary fibroblasts. The lower dermal fibroblast progenitor (PDGFRa, Blimp1−, Dlk1) generates the lower reticular fibroblasts and intradermal adipocytes/dermal white adipose tissues [40,50]. Reticular fibroblasts have a lower density and are biased for collagen I over collagen III production. Moreover, after an injury they differentiate into myofibroblasts, which promotes wound closure and presumably scarring [50]. The formation of the appendages is directly interrelated with the papillary dermis [40]. Skin development is not complete at birth because the final full barrier formation occurs afterwards.
.../...
.