.
O P E N A C C E S S S O U R C E : International Journal of Molecular Sciences
Abstract
Physical frailty and sarcopenia (PF&S) recapitulates all the hallmarks of aging and has become a focus in geroscience. Factors spanning muscle-specific processes (e.g., mitochondrial dysfunction in skeletal myocytes) to systemic changes (e.g., inflammation and amino acid dysmetabolism) have been pinpointed as possible contributors to PF&S pathophysiology. However, the search for PF&S biomarkers allowing the early identification and tracking of the condition over time is ongoing. This is mainly due to the phenotypic heterogeneity of PF&S, its unclear pathophysiology, and the frequent superimposition of other age-related conditions. Hence, presently, the identification of PF&S relies upon clinical, functional, and imaging parameters. The adoption of multi-marker approaches (combined with multivariate modeling) has shown great potential for addressing the complexity of PF&S pathophysiology and identifying candidate biological markers. Well-designed longitudinal studies are necessary for the incorporation of reliable biomarkers into clinical practice and for unveiling novel targets that are amenable to interventions.
1. Introduction
Sarcopenia is the progressive decline in muscle mass and strength that occurs during aging [1]. This condition is a hot topic in geriatric research and a public health priority [2], as it exposes older adults to increased risk of negative health-related events (such as disability, loss of independence, institutionalization, and death) [1]. However, its phenotypic heterogeneity, the unclear pathophysiology, and the frequent superimposition of other age-related conditions hamper the study of sarcopenia as a single phenomenon [3]. As a result, an univocal operational definition of sarcopenia is still missing, as are specific biomarkers that could be used, either in clinics or in research [4]. At the clinical level, sarcopenia overlaps with frailty and the age-related decline in physiologic reserve and homeostatic capacity, which predisposes older adults to a wide range of negative health-related events—including falls, morbidity, disability, hospitalization, institutionalization, and mortality [5]. In this scenario, the recognition of physical frailty and sarcopenia (PF&S) as a new entity, and its operationalization in the Sarcopenia and Physical fRailty IN older people: multi-componenT Treatment strategies (SPRINTT) project, have set a remarkable precedent for its clinical and regulatory recognition [6].
The decline in physical function is, indeed, the most evident change that occurs during sarcopenia, and a cardinal criterion for the identification of PF&S [7,8,9,10]. Physical function refers to a construct encompassing simple single-joint (e.g., handgrip strength) and multi-joint complex movements (e.g., walking speed), and may be assessed through a large array of tests [11,12].
Changes in physical performance occur slowly across years [11,13,14,15] and show great heterogeneity among people from different socioeconomic backgrounds [14]. This implies that age- and country-specific cut-off values might be needed in order to identify physical dysfunction [16]. At the same time, when considering physical dysfunction as the ultimate outcome of muscle failure [17], numerous cellular and molecular changes may occur alongside this phenomenon.
When exploring the pathways and processes involved in PF&S pathophysiology, several factors, from muscle-specific mitochondrial dysfunction to systemic changes (e.g., inflammation and amino acid dysmetabolism), have been pinpointed [9,10,18]. It is still unclear whether these processes share common roots and how cell-based alterations spread and are detected at the systemic level, contributing to the disabling cascade that characterizes PF&S. Low-grade inflammation and amino acid dysmetabolism have recently been associated with a specific pattern of small extracellular vesicles (sEVs) of mitochondrial origin, namely mitochondrial-derived vesicles (MDVs), which are proposed to function as shuttles that allow crosstalk between biological systems [7]. However, little is known about their complex regulatory network.
A conceptual framework has recently been agreed upon for the selection of blood-borne biomarkers to verify the existence of shared “hallmarks of aging” that can be targeted to extend the health span [19]. The pathophysiology of PF&S recapitulates all the hallmarks of aging [19,20,21], which makes it a prototypical geroscience condition [22]. In this scenario, multivariate analyses of biomediators, pertaining to different domains, may enable the identification of biomarkers for PF&S that capture its pathophysiological complexity (Figure 1) [7,8,9,10,23]. The identified pathways may, eventually, be used for drug development.
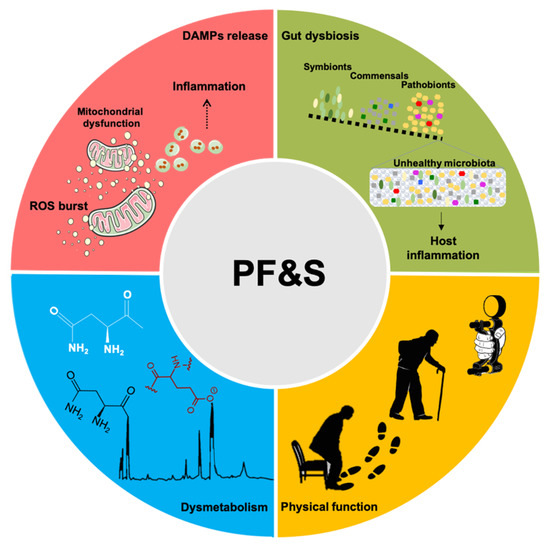
Figure 1. Schematic representation of the main pathophysiological pathways contributing to physical frailty and sarcopenia (PF&S) (i.e., inflammation, gut dysbiosis, declines in physical function, and dysmetabolism), and related biomarkers. Abbreviations: DAMPs, damage-associated molecular patterns; ROS, reactive oxygen species.
Here, we will provide an overview of imaging, functional, and biological markers currently available for PF&S. We will also illustrate the prospect of exploiting specific biological processes to identify new biomarkers for the condition and to develop personalized interventions.
2. Imaging and Functional Markers
When Irwin Rosenberg prompted health professionals to pay serious attention to sarcopenia [24], he referred exclusively to age-related muscle atrophy, while changes in physical function and mobility were seen as possible secondary outcomes. Indeed, sarcopenia and muscle atrophy were synonyms until the 2000s [25,26,27], after which findings from observational studies indicated that losses in physical function occurred earlier, progressed more rapidly, and were better predictors of negative health-related events than a decline in muscle mass [13,28]. Indeed, the recognition of declining physical performance as a core feature of muscle aging has prompted the appraisal of sarcopenia as a composite condition that encompasses quantitative (mass) and qualitative (strength/function) muscular domains [29,30].
Body imaging techniques (e.g., dual energy x-ray absorptiometry (DXA), computed tomography (CT), and magnetic resonance imaging (MRI)) are popular tools for the quantification of muscle or lean body mass [31]. However, all of them show notable pitfalls. Indeed, the results of DXA are heavily influenced by body thickness, hydration status, and extracellular fluid accumulation. Furthermore, DXA is unable to measure intramuscular adipose tissue [31]. On the other hand, the large-scale implementation of CT and MRI is hampered by high costs, technological complexity, and space requirements [31]. CT also exposes the test person to non-negligible doses of ionizing radiation.
The creatine (methyl-d3) dilution (D3-creatine) method is a recently developed approach that allows the accurate quantification of whole-body muscle mass [32]. The method relies on the irreversible conversion of creatine to creatinine, and its excretion in urine. The enrichment of urine D3-creatinine allows estimating the total body creatine pool size as a proxy for the whole-body skeletal muscle mass. This method involves the oral administration of stable isotope-labeled creatine (D3-creatine), followed by the collection of a single fasted urine sample 48–96 h later. The sample is assayed for creatine and creatinine (deuterated and unlabeled) via liquid chromatography mass spectrometry (LC-MS) [33,34,35]. By means of an algorithm, this method allows us to calculate the total body creatine pool size and the muscle mass from D3-creatinine enrichment in urine [33,34,35]. Estimates of the total body muscle mass, obtained by this method, show remarkable concordance with the whole-body MRI scans [33,34,35]. Differently from the DXA, the muscle mass quantified by the D3-creatine dilution method is strongly correlated with physical performance, and predicts incidents of falls and functional limitations [36,37,38,39].
Physical function starts declining around the third decade of life, with a steeper decrease beyond the age of 50, which suggests that the initial deflection of physical performance might be an early predictor of sarcopenia [11,13,14,15]. However, physical performance refers to a wide construct involving several components, including (but not limited to) muscle strength, power, and mobility. Therefore, several physical abilities are legitimate functional biomarkers of sarcopenia. The identification of specific components of physical function that, more so than others, predict sarcopenia is particularly challenging, especially because knowledge of this condition has grown considerably in the last two decades, as reflected by changes in its conceptual framework and operational definitions [30,40,41].
Muscle strength refers to the amount of force generated by a dynamic muscle contraction [42,43]. Isometric handgrip strength (IHG) and the five-time sit-to-stand test (5×STS) are two simple, inexpensive and quick tests used to assess upper and lower limb muscle strength, respectively, in different settings, including the community, hospitals, and nursing homes [4,29,30]. Cross-sectional [44,45,46,47,48] and prospective studies [44,47,49,50,51,52] have reported that low IHG is significantly associated with poor physical performance, mobility impairment, and disability. Notable findings by Rantanen et al. [52] reported that men with low IHG during midlife were at higher risk of developing physical dysfunction 25 years later [52]. Although several studies support the hypothesis that IHG might predict physical dysfunction (and, likely, also sarcopenia), there is also evidence indicating that IHG is not associated with lower extremity disability [53]. Therefore, the combination of upper and lower limb muscle strength testing might be necessary for adequately framing sarcopenia. The 5×STS is associated with many physical performance tests [54], progressive disability [53], and has been reported as a predictor of sarcopenia in older Brazilian women [55]. However, larger studies, also including older men, are needed to confirm and expand these initial findings.
Muscle power—the capacity to generate force in a short time interval—declines earlier in life and at a faster rate, while presenting a higher degree of association with some mobility tasks than muscle strength [13,56,57]. For instance, results from the InCHIANTI (Invecchiare in Chianti) study indicated that women aged between 50 and 60 years had a 20–30% reduction of lower limb muscle strength, while muscle power was reduced by ~50% [13]. Lower limb muscle power was also indicated as a better discriminator of poor mobility than upper and lower limb muscle strength [13,57].
In an attempt to empower the discriminatory capacity of physical function as a marker of PF&S, researchers have proposed combinations of physical tests that support the assessment of different physical abilities. The short physical performance battery (SPPB) provides a single score based on a person’s performance in balance tests, gait speed, and 5×STS [58]. In the seminal study by Guralnik et al. [58], the authors observed that people with low SPPB scores were almost five times more likely to develop disability in a short time interval. These findings were confirmed by a recent systematic review and meta-analysis [59] that indicated that lower SPPB was associated with worsening activities of daily living (ADL) and instrumental ADL.
Poor physical function is associated with chronic low-grade inflammation and antioxidant markers in older adults [60,61,62,63,64], thus suggesting that physical dysfunction is likely mediated by cellular mechanisms that, in combination with functional assessment, may be more informative than physical function alone.
3. Inflammation-Related Biomarkers
Dysregulation of the cytokine network is a major driver of aging and related conditions [65,66,67,68]. However, the inclusion of inflammatory markers in clinical practice, as a tool for identifying specific conditions, is far from being reached [19]. A core inflammatory profile with gender-specific signatures has been identified in the context of PF&S as composed by higher levels of C-reactive protein and lower concentrations of myeloperoxidase (MPO), interleukin (IL) 8, monocyte chemoattractant protein 1, and platelet-derived growth factor-BB (PDGF-BB). This set of mediators includes markers related to immunosenescence [69,70], micronutrient intake imbalance [71,72], and impaired muscle regeneration, in response to specific stimuli, such as oxidative stress [73,74,75,76,77]. Senescent cells face morphological and functional reshaping, manifested by a senescence-associated secretory phenotype (SASP) [78]. The SASP fingerprint is composed of ILs, chemokines, growth factors, proteases, and extracellular matrix components [78]. The release of these SASP factors induces perturbations in the local microenvironment through autocrine and paracrine signals, in order to prevent proliferation of damaged cells, as well as enabling the recruitment of immune cells and promoting tissue repair [79,80]. Age-related decline in cell quality control systems may induce insufficient clearance of senescent cells and support systemic inflammation, via the overproduction of SASP-related pro-inflammatory cytokines (e.g., IL1β, IL6, and IL8) [81].
A pro-disability effect has long been attributed to the chronic low-grade inflammation observed during aging, referred to as inflamm-aging [68,70,82]. Indeed, higher levels of pro-inflammatory cytokines have been associated with muscle wasting and reduced physical performance [70,83]. This inflammatory response, void of infectious agents and named “sterile inflammation”, is part of the innate immune response triggered by misplaced cellular components [84,85], which are rooted into the “danger theory” of inflammation [86]. According to this theory, the accumulation of damage-associated molecular patterns (DAMPs), and their release from injured cells into the circulation, triggers inflammation via caspase-1 activation and the release of proinflammatory cytokines [87]. Among DAMP molecules, those of mitochondrial origin, in light of their bacterial ancestry, are thought to contribute substantially to inflamm-aging by interacting with Toll-like receptors, NLR family pyrin domain containing 3 inflammasome activation, and cytosolic DNA sensing by the guanosine monophosphate–adenosine monophosphate (GMP–AMP) synthase–stimulator of interferon gene systems [88,89,90].
The mitochondrial involvement in the crosstalk between chronic inflammation and muscle wasting is not surprising given the central role of this organelle in skeletal myocyte viability. Mitochondria are highly interconnected organelles and form a dynamic network that operates via intra-mitochondrial contacts (as well as with the endoplasmic reticulum, lysosomes, and the actin cytoskeleton) to guarantee organelle homeostasis [91,92]. Recently, mitochondrial tubular protrusions, named mitochondrial nanotunnels, have also been identified as additional structures for mitochondrial interconnections, especially in mitochondria immobilized within post-mitotic tissues (e.g., skeletal muscle and cardiac tissue) [92]. These resident organelles are structurally limited in their fusion, and thus may communicate over long distances via nanotunnels [92]. Finally, Golgi-derived vesicles have been identified to participate in mitochondrial dynamics and are newly described contact sites involved in mitochondrial homeostasis [93]. However, the networking ability of mitochondria may be a double-edged sword. Indeed, it is unclear where the boundary lies between the protective role of the inflammation-derived circulating DAMPs and the detrimental effect of over-reactive inflammation. Severe mitochondrial damage and abnormal autophagosome formation were found in the skeletal muscle of IL10-null mice (IL10tm/tm), a rodent model of chronic inflammation and frailty [94]. Among mitochondrial DAMPs, circulating mitochondrial DNA (mtDNA) released from damaged organelles is a prominent candidate, linking inflammation with muscle decay [85]. Recent findings by our group support the hypothesis of mitochondrial impairment among the underlying pathogenetic mechanisms of sarcopenia [18]. In particular, as a result of failing quality control systems [91,95,96,97], the release of oxidized cell-free mtDNA and other mitochondrial components within MDVs have been associated with systemic inflammation in older adults with PF&S [7].
The characterization of the composition of exosomes/EVs released by senescent cells (eSASP) has revealed a specific EV SASP signature [98] from which it has been possible to track down their originating cells [99,100]. Therefore, EVs represent a unique tool for capturing the regulatory network of complex conditions and for the identification of cell- and stressor-specific biomarkers. The dissection of these pathways may provide relevant insights into the role played by inflammation in the disabling cascade of PF&S, allowing for the design of personalized treatment strategies.
.../...
.
Edited by Engadin, 26 August 2020 - 11:54 AM.